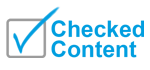
Thiamine
Background Information
SOS Children offer a complete download of this selection for schools for use on schools intranets. A good way to help other children is by sponsoring a child
Thiamine | |
---|---|
![]() |
|
![]() |
|
2-[3-[(4-amino-2-methyl- pyrimidin-5-yl)methyl]- 4-methyl-thiazol-5-yl] ethanol |
|
Identifiers | |
CAS number | 59-43-8 |
PubChem | 1130 |
MeSH | Thiamin |
Jmol-3D images | Image 1 |
SMILES
|
|
Properties | |
Molecular formula | C12H17N4OS+ |
Molar mass | 265.356 |
Melting point |
248-260 °C (hydrochloride salt) |
Except where noted otherwise, data are given for materials in their standard state (at 25 °C, 100 kPa) | |
Infobox references |
Thiamin or thiamine, also known as vitamin B1 and aneurine hydrochloride, is one of the B vitamins. It is colorless chemical compound with a chemical formula C12H17N4OS. It is soluble in water, methanol, and glycerol and practically insoluble in acetone, ether, chloroform, and benzene. Thiamin decomposes if heated. Its chemical structure contains a pyrimidine ring and a thiazole ring.
Thiamin is essential for neural function and carbohydrate metabolism. A severe thiamin deficiency results in Beriberi which is a nerve and heart disease. In less severe deficiency, nonspecific signs include malaise, weight loss, irritability and confusion.
History
Thiamin was first discovered in 1910 by Umetaro Suzuki in Japan when researching how rice bran cured patients of beriberi. He named it aberic acid (later oryzanin). He did not determine its chemical composition, nor that it was an amine. It was first crystallized by Jansen and Donath in 1926 (they named it aneurin, for antineuritic vitamin). Its chemical composition and synthesis was finally reported by Robert R. Williams in 1935. He also coined the current name for it, thiamine.
Antagonists
Thiamin in foods can be degraded in a variety of ways. Sulfites, which are added to foods usually as a preservative, will attack thiamin at the methylene bridge in the structure, cleaving the pyrimidine ring from the thiazole ring. The rate of this reaction is increased under acidic conditions. Thiamin can also be degraded by thiaminases. Some thiaminases are produced by bacteria. Bacterial thiaminases are cell surface enzymes that must dissociate from the membrane before being activated. The dissociation can occur in ruminants under acidotic conditions. Rumen bacteria also reduce sulfate to sulfite, therefore high dietary intakes of sulfate can have thiamin-antagonistic activities.
Plant thiamin antagonists are heat stable and occur as both the ortho and para hydroxyphenols. Some examples of these antagonists are caffeic acid, chlorogenic acid and tannic acid. These compounds interact with the thiamin to oxidize the thiazole ring, thus rendering it unable to be absorbed. Two flavonoids, quercetin and rutin, have also been implicated as thiamin antagonists.
Absorption
Thiamin is released by the action of phosphatase and pyrophosphatase in the upper small intestine. At low concentrations the process is carrier mediated and at higher concentrations, absorption occurs via passive diffusion. Active transport is greatest in the jejunum and ileum. The cells of the intestinal mucosa have thiamin pyrophosphokinase activity, but it is unclear whether the enzyme is linked to active absorption. The majority of thiamin present in the intestine is in the phosphorylated form, but when thiamine arrives on the serosal side of the intestine it is often in the free form. The uptake of thiamine by the mucosal cell is likely coupled in some way to its phosphorylation/dephosphorylation. On the serosal side of the intestine, evidence has shown that discharge of the vitamin by those cells is dependent on Na+-dependent ATPase.
Transport
Bound to serum proteins
The majority of thiamin in serum is bound to proteins, mainly albumin. Approximately 90% of total thiamin in blood is in erythrocytes. A specific binding protein called thiamin-binding protein (TBP) has been identified in rat serum and is believed to be a hormonally regulated carrier protein that is important for tissue distribution of thiamin.
Cellular uptake
Uptake of thiamin by cells of the blood and other tissues occurs via active transport. About 80% of intracellular thiamin is phosphorylated and most is bound to proteins. In some tissues, thiamin uptake and secretion appears to be mediated by a soluble thiamin transporter that is dependent on Na+ and a transcellular proton gradient. The highest concentration of the transporter have been found in skeletal muscle, heart, and placenta.
Tissue Distribution
Human storage of thiamin is about 25 to 30 mg with the greatest concentrations in skeletal muscle, heart, brain, liver, and kidneys. Thiamin monophosphate(TMP) and free thiamin is present in plasma, milk, cerebrospinal fluid, and likely all extracellular fluids. Unlike the highly phosphorylated forms of thiamin, TMP and free thiamin are capable of crossing cell membranes. Thiamin contents in human tissues are less than those of other species.
Deficiency
Systemic thiamin deficiency can lead to myriad problems including neurodegeneration, wasting and death. A lack of thiamin can be caused by malnutrition, alcoholism, a diet high in thiaminase-rich foods (raw freshwater fish, raw shellfish, ferns) and/or foods high in anti-thiamine factors (tea, coffee, betel nuts).
Well-known syndromes caused by thiamin deficiency include Wernicke-Korsakoff syndrome and beriberi, diseases also common with chronic alcoholism.
Polioencephalomalacia (PEM), is the most common thiamin deficiency disorder in young ruminant and nonruminant animals. Symptoms of PEM include a profuse, but transient diarrhea, listlessness, circling movements, star gazing or opisthotonus (head drawn back over neck), and muscle tremors.
It is thought that many people with diabetes have a deficiency of thiamin and that this may be linked to some of the complications that can occur.
Alcoholic Brain Disease
Thiamin and thiamin-using enzymes are present in all cells of the body, thus, a thiamin deficiency would seem to adversely affect all of the organ systems. However, the nervous system (and heart) shows particular sensitivity to the effects of a thiamin deficiency at the cellular level.
Nerve cells and other supporting cells (such as glial cells) of the nervous system require thiamin. Examples of neurologic disorders that are linked to alcohol abuse include Wernicke’s Encephalopathy ( Wernicke-Korsakoff syndrome) and Korsakoff’s psychosis (alcohol amnestic disorder) as well as varying degrees of cognitive impairment.
How does alcoholism induce thiamin deficiency? The enzymes transketolase, pyruvate dehydrogenase (PDH) and alpha-ketoglutarate dehydrogenase (α-KGDH) all require thiamin as a cofactor in order to function in carbohydrate metabolism. Therefore, a thiamin deficiency would be detrimental to the functionality of these enzymes. Transketolase is important in the pentose phosphate pathway. PDH and α-KGDH function in biochemical pathways that result in the generation of adenosine triphosphate (ATP), which is a major form of energy for the cell. PDH is also needed for the production of acetylcholine, a neurotransmitter, and for myelin synthesis. During metabolism, PDH determines whether the process is aerobic or anaerobic, and α-KGDH is responsible for determining the rate of the citric acid cycle.
What are the mechanisms of alcohol-induced thiamin deficiency? 1) Inadequate nutritional intake: Alcoholics tend to intake less than the recommended amount of thiamin, however it is also seen that others have an extremely high level of free thiamin, suggesting an inability of these individuals to convert thiamin to the biologically active, phosphorylated form. 2) Decreased uptake of thiamin from the GI tract: Active transport of thiamin into the enterocyte occurs mostly in conditions of low thiamin concentration. The absorption is disturbed during acute alcohol exposure as illustrated by less thiamin being converted into the phosphate-containing form, suggesting a dysfunction of the enzyme responsible for this transformation: thiamin diphosphokinase. 3) Impaired thiamin utilization: Magnesium, which is required for the binding of thiamin to thiamin-using enzymes within the cell, is also deficient due to chronic alcohol consumption. The inefficient utilization of any thiamin that does reach the cells will further exacerbate the thiamin deficiency.
Following improved nutrition and the removal of alcohol consumption, some impairments linked with thiamin deficiency are reversed; particularly poor brain functionality.
Diagnostic testing
A positive diagnosis test for thiamine deficiency can be ascertained by measuring the activity of the enzyme transketolase in erythrocytes. Thiamine can also be seen directly in whole blood following the conversion of thiamine to a fluorescent thiochrome derivative. However, this test may not reveal the deficiency in diabetic patients.
Thiamine phosphate derivatives
There are four known natural thiamine phosphate derivatives: thiamine monophosphate (ThMP), thiamine diphosphate (ThDP) or thiamine pyrophosphate (TPP), thiamine triphosphate (ThTP), and the recently discovered adenosine thiamine triphosphate (AThTP).
Thiamine pyrophosphate
Thiamine pyrophosphate (TPP), also known as thiamin diphosphate (ThDP), and cocarboxylase is a coenzyme for several enzymes that catalyze the dehydrogenation ( decarboxylation and subsequent conjugation to Coenzyme A) of alpha-keto acids. Examples include:
- In mammals:
- pyruvate dehydrogenase and α-ketoglutarate dehydrogenase ( metabolism of carbohydrates)
- branched-chain alpha-keto acid dehydrogenase
- 2-hydroxyphytanoyl-CoA lyase
- transketolase (functions in the pentose phosphate pathway to synthesize NADPH and the pentose sugars deoxyribose and ribose )
TPP is synthesized by the enzyme thiamin pyrophosphokinase, which requires free thiamin, magnesium, and adenosine triphosphate.
Thiamine triphosphate
Thiamine triphosphate (ThTP) was long considered a specific neuroactive form of thiamin. However, recently it was shown that ThTP exists in bacteria, fungi, plants and animals suggesting a much more general cellular role. In particular in E. coli it seems to play a role in response to amino acid starvation.
Adenosine thiamine triphosphate
Adenosine thiamine triphosphate (AThTP) or thiaminylated adenosine triphosphate has recently been discovered in Escherichia coli where it accumulates as a result of carbon starvation. In E. coli, AThTP may account for up to 20 % of total thiamin. It also exists in lesser amounts in yeast, roots of higher plants and animal tissues.
Genetic diseases
Genetic diseases of thiamin transport are rare but serious. Thiamin Responsive Megaloblastic Anaemia with diabetes mellitus and sensorineural deafness (TRMA) is an autosomal recessive disorder caused by mutations in the gene SLC19A2, a high affinity thiamine transporter. TRMA patients do not show signs of systemic thiamin deficiency, suggesting redundancy in the thiamin transport system. This has led to the discovery of a second high affinity thiamin transporter, SLC19A3.
Research
High doses
The RDA in most countries is set at about 1.4 mg. However, tests on volunteers at daily doses of about 50 mg have claimed an increase in mental acuity.
Thiamin as an insect repellent
Some studies suggest that taking thiamin 25 to 50 mg three times per day is effective in reducing mosquito bites. A large intake of thiamin produces a skin odor that is not detectable by humans, but is disagreeable to female mosquitoes. Thiamin takes more than 2 weeks before the odour fully saturates the skin. With the advances in topical preparations there is an increasing number of thiamin based repellent products. There is anecdotal evidence of thiamin products being effective in the field (Australia, US and Canada), but one study found thiamin had no effect.
Autism
A 2002 pilot study administered thiamin tetrahydrofurfuryl disulfide (TTFD) rectally to ten autism spectrum children, and found beneficial clinical effect in eight. This study has not been replicated and a 2006 review of thiamin by the same author did not mention thiamin's possible effect on autism.